Electron Trajectories in Scanning Field-Emission Microscopy
The Scanning Field Emission Microscopy (SFEM) is a novel technology similar to the better known Scanning Tunneling Microscopy (STM). In STM, electrons are exchanged between the outermost atom of a sharp tip and the outermost atom of a target over sub-nanometer distances by means of the quantum mechanical tunnel effect. When the tip is scanned parallel to the surface, the tunneling current can be recorded and used to map the surface topography at atomic scale. In SFEM, the distance between tip and target is larger than 4 nm and the voltage is typically in the range between 20 and 50 V.
This paper reports on a numerical study of some aspects of the transport of electrons between tip-apex and target: i) the electric field assisted tunneling of electrons from the metallic side of the tip into the vacuum side of the tip, ii) the transport of the resulting primary electron beam to the target, and iii) the emission of secondary electrons from the target, which might escape the tip-target junction, reach the macroscopic environment and become available for further analysis. The energy spectrum of the secondary electrons [2] indicates that both low energy and elastic electrons escape the junction. In addition, secondary electrons emitted from a magnetic target turn out to be spin polarized. These properties can be used for chemical mapping [2] or magnetic mapping [1] with nanometer spatial resolution.
The trajectories of the electrons are computed with the AC/DC and Particle Tracing Modules of COMSOL Multiphysics®. The first simulations consider the primary beam of electrons generated at the tip-apex. The width of the primary beam turns out to be of the order of the tip-target distance. This result contradicts the extraordinary small lateral spatial resolution detected in a different mode of SFEM, based on detection of the secondary electrons and leave this observation unexplained [3]. The trajectories of the secondary electrons emerging from the target are computed by implementing a multiscale coupled simulation consisting of a Junction-Component (sub-nanometer resolution) and a System-Component which models the experimental setup (dimensions of many tens of cm).
Important results are that most secondary electrons return to the target within few 10 nm from their spot of generation. Only few of them reach the detector, and most favored are those with the highest energy. In addition, we found that positioning the tip close to the very edge of the target increases substantially the secondary electron yield at the detector site. This edge-effect was observed also experimentally and turned out to be the key element for efficient spin detection [1].
[1] Pietro, L. G. D. Field Emission Scanning Tunnelling Microscopy with Spin Polarisation Analysis (ETH Zurich, Diss. No. 23714, 2016).
[2] Zanin, D. A. Fundamental aspects of scanning field-emission microscopy and some similarities with critical phenomena (ETH Zurich, Diss. No. 24169, 2017).
[3] Kyritsakis, A. & Xanthakis, J. Beam spot diameter of the near-field scanning electron microscopy. Ultramicroscopy 125, 24–28. issn: 0304-3991 (2013).
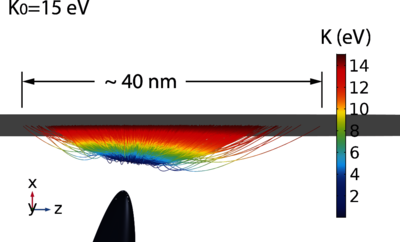
Herunterladen
- cabrera_presentation.pdf - 1.2MB
- cabrera_abstract.pdf - 0.1MB